
However, the SW launched by the feed can be always assumed to be cylindrical and azimuthally symmetric, as the one provided by a monopole. The feeding system might also be more complex, e.g., a hat-feed excited by a TM 01 circular waveguide, to cover a larger bandwidth or to improve the efficiency of SW excitation. This allows an impedance matching as well as the maximization of the SW power launched on the MTS.
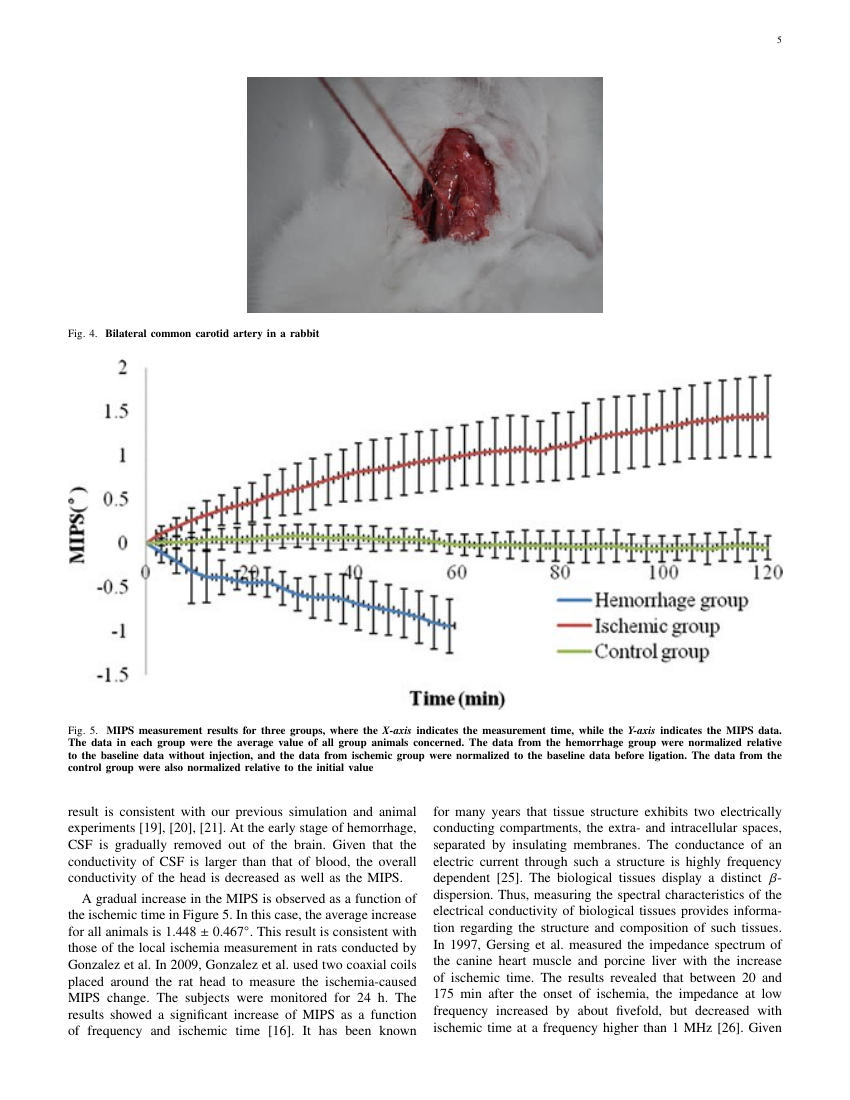
#Ieee transactions on antenna and wave propagation Patch#
Usually, the monopole passes through the center of an annular patch loaded with an annular slot. The SW launcher is simply a coaxially fed vertical monopole and it is located at the center of the circular aperture (see Fig. Despite its discretized nature, the impedance BC formed by the patch texture can be modelled within an excellent approximation as a continuous sheet of anisotropic impedance BC, which supports and modifies the SW propagation. Usually, the lattice is of Cartesian type, but one could also exploit hexagonal lattices 33.įigure 1(a–f) shows examples of pixels we used to implement the MTS. The pixels are arranged in a regular lattice with elementary cell size in the range λ/10-λ/5. The MTS constitutive elements behave like pixels in a black and white printed image, whose gray scale is realized by changing the dimension of the elements inside a regular lattice.

Hence, the interacting SW sees the interface impedance BC as a continuum. At the frequency of operation, the elements that implement the impedance BCs are small in terms of the wavelength (typically, between λ/5 and λ/10, with λ being the free-space wavelength). The MTSs use elements whose geometries gradually change from cell-to-cell. Implementation of MTS structures able to simultaneously support TM and TE radiating modes have been shown in 33. Accurate control of the beam polarization has been demonstrated in 16, 30 syntheses of shaped apertures have been proposed in 31, 32. Also, the possibility of obtaining multibeam radiation from a single aperture has been displayed, by coupling a MTS and Luneburg lenses in 28 or in 29. In 4, 27, Huygens MTSs have been proposed to acquiring additional degrees of freedom in the control of the radiating field. Challenging designs have been also made possible through the evolution of MTSs modeling 26, in the perspective of beam scanning. These attractive features, combined with the possibility of a fine control and shape tailoring of the aperture field, result in a number of interesting antenna devices recently proposed in literature.
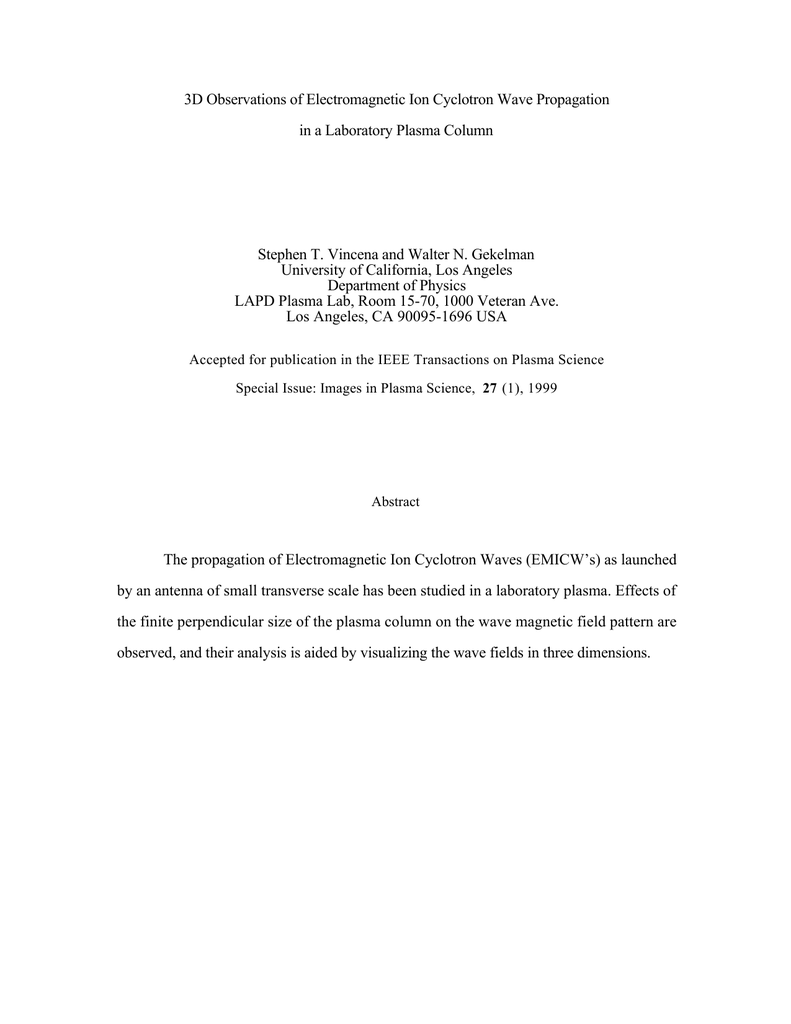
Moreover, the feeding element is embedded in the MTS plane, so that one can avoid external protruding or backing feed arrangements or (sub-) reflectors. The typical MTS structure presents a low profile, weight, and complexity, and can be manufactured with standard printed circuit board techniques. When the surface wave (SW) interacts with modulated, locally periodic boundary conditions (BCs) imposed by a MTS, it allows one to reproduce a desired aperture field radiating through a leaky wave (LW) effect 24, 25. Alternatively, at submillimeter wavelengths, MTSs can be constituted by a dense distribution of metallic pins on a ground plane 22, 23. In microwave antennas applications, MTSs are usually formed by a regular texture of small elements printed on a grounded slab with or without shorting vias 10, 11, 12, 13, 14, 15, 16, 17, 18, 19, 20, 21. MTS technology has received considerable attention by the scientific community and has been employed in a number of applications a recent overview can be found in 9. They can be designed to achieve unusual reflection/transmission properties of space waves and/or to modify the dispersion properties of surface/guided waves 1, 2, 3, 4, 5, 6, 7, 8. Metasurfaces (MTSs) are thin artificial layers consisting of periodic arrangements of small inclusions in a dielectric host medium.
